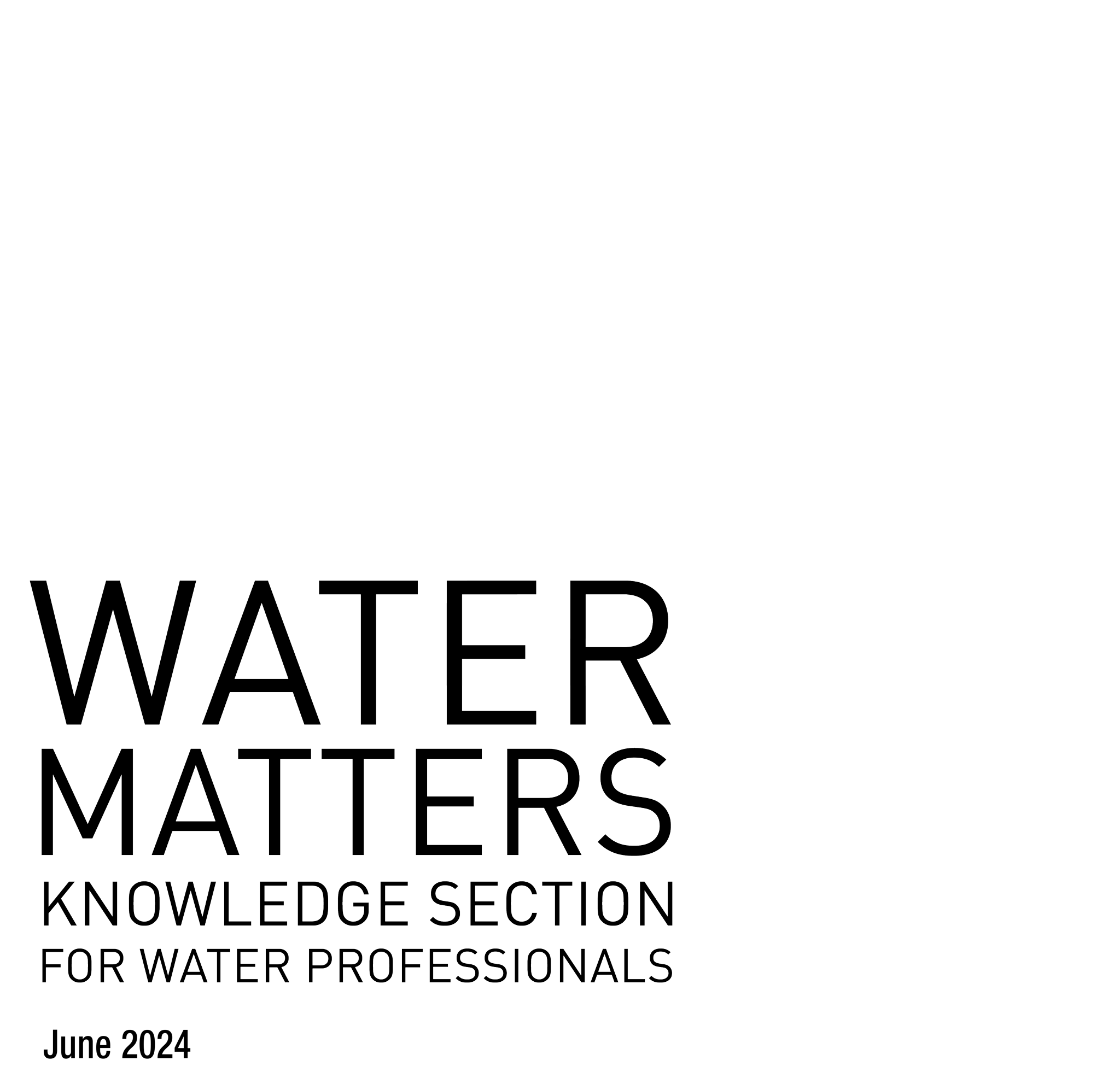
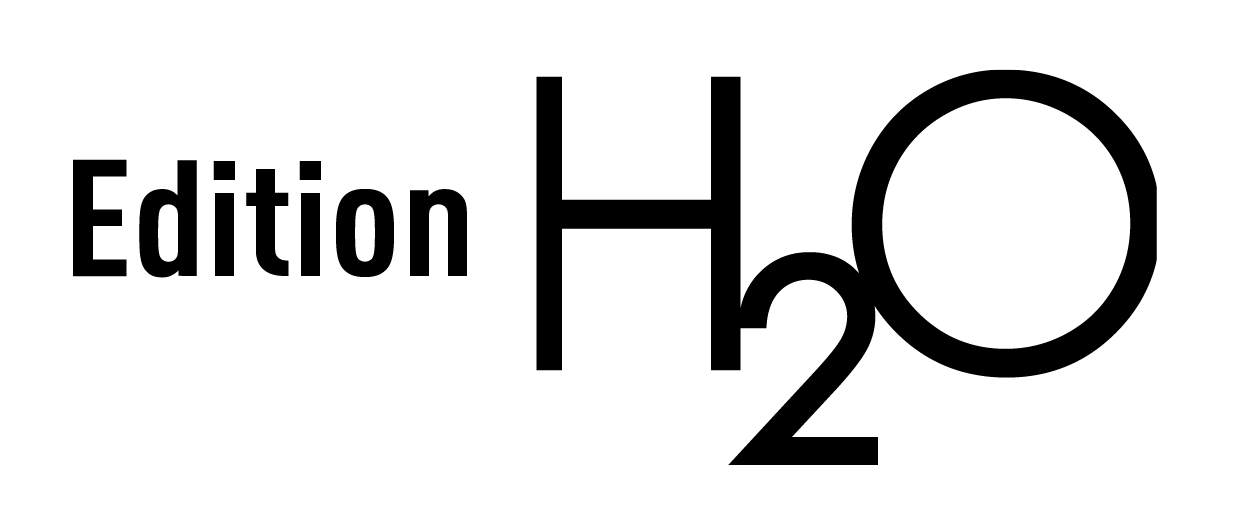
You are reading the eighteenth edition of Water Matters, the knowledge magazine of the Dutch specialist journal H2O. You will find eight articles on a variety of topics, written by water professionals based on solid research.
The editorial board, consisting of experts from the sector, made a selection based on a clear relationship with daily practice in the water sector, which is the purpose of Water Matters. Research, results and findings form the basis for articles that describe new knowledge, insights and technologies with a view to practical application.
In this edition of Water Matters, you will read how nutrient concentrations have dropped significantly and aquatic plants have increased sharply in Bleiswijkse Zoom, a recreational area in the municipality of Bleiswijk. The water and green area was plagued by blue-green algae for years, but now almost meets the targets of the European Water Framework Directive. Thanks to an integrated plan drawn up on the basis of a system analysis using the key ecological factors.
Furthermore, you will find articles on:
- the development of a digital twin for the Maeslant barrier (storm surge barrier at Hoek van Holland)
- a 'passage check' to examine and improve the functioning of fish passages
- the ecological state of Dutch canals: from open sewer to urban oasis (canals with more biodiversity emit less greenhouse gases)
- the effect of fast-growing non-native floating pennywort on nature-friendly banks and the impact of illicit connections in separate wastewater sewer systems on antibiotic-resistant surface water
- an inventory of PFAS in surface water, groundwater and drinking water in the Netherlands
- the Lake Report, a forecast of safe bathing water.
Water Matters, like the magazine H2O, is an initiative of Royal Dutch Water Network (KNW), the independent knowledge network for and by Dutch water professionals. KNW members receive Water Matters twice a year free of charge as a supplement to their journal H2O.
The publication of Water Matters is made possible by leading players in the Dutch water sector. These Founding Partners are Deltares, KWR Watercycle Research Institute, Royal HaskoningDHV and Stichting Toegepast Onderzoek Waterbeheer (STOWA). With the publication of Water Matters, the participating institutions aim to make new, applicable water knowledge accessible.
The English-language articles can be shared from the digital magazine on H2O-online. Furthermore, articles from previous editions can be found on the website.
Would you like to respond? Let us know via redactie@H2O-media.nl.
Monique Bekkenutte
Publisher (Royal Dutch Water Network)
Huib de Vriend
Chairman of the editorial board Water Matters
Dozens of pumping stations and locks along the coast have been equipped with fish passages to allow glass eels (juvenile eels) and three-spined sticklebacks to travel inland from the sea. Constructing fish passages is expensive, and they often do not work optimally. The 'Fish Passage Check' makes it possible to assess the effectiveness of fish passages and how they can be improved.
Glass eels are 'weak swimmers' that struggle to reach fresh inland waters from the sea in spring, due to the many locks and pumping stations. To learn more about this and identify bottlenecks, RAVON launched the Fish Passage Check in 2017: a standardised method of determining the effectiveness of fish passages for glass eels (and three-spined sticklebacks). Since then, we have surveyed nearly 20 sites. This article is about the Scheveningen case study.
In Scheveningen, water is drained from the Delflandse Boezem to the North Sea via the Schoute Pumping Station. The Keersluis lock, which originally always remained closed except when draining water, is located another 550 metres closer to the sea. In 2011, a lock chamber located under the pumping station was commissioned as a 'fish sluice'. During the fish migration season, the following would occur:
• At night, when the tide was just right, the pumping station would run with the Keersluis lock temporarily open, so that fish could reach the pumping station.
• After that, the Keersluis lock would close and the water level between the pumping station and the Keersluis lock would be raised by running the pumping station.
• The lock chamber would then open once for 20 minutes, during which time water, hopefully with migratory fish, would flow back to the ‘boezem’.
The effectiveness of this passage for glass eels was investigated in 2017[1]. This was the first time VIE (visible implant elastomer) tags were used in the Netherlands: glass eels were given a subcutaneous colour tag with a fluorescent elastomer. Marked glass eels were tracked using different (re)capture methods both in front of and behind the Keersluis. Five groups of glass eels were tagged, 1,322 individuals in total.
Based on the catches, it was calculated that around 94,000 glass eels arrived at the complex in 2017. The migration into fresh water was not efficient; in eight days, less than 1 per cent entered the fyke on the inland side (the ‘boezem’ side) of the fish passage. Glass eels accumulated before the Keersluis lock, and densities elsewhere were low.
The results from 2017 led to several improvements. The first measure was to keep the Keersluis lock open as much as possible. This can be done outside the storm season (15 March–15 October) and when water levels and salinity allow. An attraction flow pump was also installed in the lock chamber, pumping water from the ‘boezem’ into the lock chamber, up to a slide at the halfway point.
In addition, automation was updated. Every day at dusk, one hour of pumping is carried out in advance with a single pumping-station pump (about 300 m3/min). A one-hour cycle then begins:
• The lock chamber opens on the sea-facing side, and the attraction flow pump starts pumping (max 6.75 m3/min at 1,450 rpm). Fresh water flows out towards the (open) Keersluis lock, and fish can swim into the lock chamber against the current.
• After 30 minutes, the attraction flow pump stops and the lock chamber closes again.
• Then the slide in the lock chamber goes up for 30 minutes, the lock chamber empties towards the ‘boezem’ and the fish can reach the freshwater.
• This cycle is repeated until sunrise.
The new layout was ready in early 2020. Immediately after the start of the migration season, volunteers noticed changes in their weekly counts (as part of ‘Samen voor de Aal’ (‘Together for Eels’), since 2015). At the Keersluis lock, they caught far fewer glass eels, while they caught many more at the Schoute Pumping Station. In short, glass eels were better able to swim to the pumping station.
In 2021, we conducted detailed research on the inland migration of glass eels through the 'Fish Passage Check'. The aim was to determine the 'supply' of glass eels, evaluate the passage efficiency in the new situation and determine possible follow-up steps.
At different times and locations, 24 unique VIE-tagged groups were released, totalling 2,762 glass eels. Of these, 452 were released on the sea-facing side of the Keersluis lock, 2,060 on the sea-facing side of the Schoute Pumping Station and 250 on the ‘boezem’ side in the fish passage's lock chamber. Both at the pumping station and at the Keersluis lock, on the sea-facing side, a glass eel collector was deployed – a kind of 'hoover' that filters water and thus catches glass eels. Moreover, both sites were intensively lift net fished by volunteers. A glass eel detector was also deployed on the sea-facing side of the pumping station: a ramp (crawling gutter) with climbing substrate and an attraction flow in which glass eels actively climb. The ‘boezem’ side of the fish passage was fitted with a large fine-mesh fyke to catch transiting glass eels. To interpret the functioning of the fish passage and observe behaviour, we took underwater images and measured flow velocities. Records of settings and running times of the pumping station, the Keersluis lock and the fish passage were also read out.
Mark-recapture statistics showed that some 125,000 glass eels arrived in Scheveningen in 2021. The Keersluis lock, since it is usually open, is no longer an obstacle. At the pumping station, glass eels were accumulating, which was also reflected in the catches of volunteers. Migration throughout the entire complex was slow, but much more successful in the first eight days than in 2017: about 7.2 per cent compared to 0.8 per cent in 2017, based on a direct comparison of tagged groups that were caught/released at the Keersluis lock and migrated through to the ‘boezem’. Ultimately, about 29 per cent of all glass eels successfully migrated through the fish passage.
Underwater images and measurements of flow velocities show that glass eels were physically able to pass well but experienced problems with their orientation due to changing flow patterns during the lock cycle.
Many glass eels 'disappeared' in the fish passage. This most likely happened due to suction in the attraction flow pump (located in the lock chamber), leading to mortality. Finally, the set cycle was found to be malfunctioning. Only 2 cycles of twice 30 minutes per night usually took place (sometimes even just 1), and there were frequent failures that meant the fish passage did not work overnight at all.
The main recommendations are to move the intake of the attraction flow pump outside the fish passage and to run the fish migration cycle more frequently (eight times per night). This will potentially increase the fish passage efficiency for glass eels from 29 to 75 per cent, according to calculations. Further 'fine-tuning' is also possible: placing the attraction flow closer to the fish migration gate, increasing the opening of the lock chamber and 'opening' the fish passage for longer in the season. When implementing these measures, it is again important to carefully test the effects.
The Fish Passage Check in Scheveningen shows that attention to fish migration is badly needed even after the construction of a fish passage. These findings are not isolated, with major improvement possible at almost all fish passages studied. On average, for glass eels, only about 25 per cent passed successfully, with the worst-performing passage passing less than one per cent and the best exceeding 80 per cent. Similar results apply to three-spined sticklebacks, which are also weak swimmers.
Thanks to numerous studies, a variety of bottlenecks have been identified in recent years. These include both design flaws (such as a collection trough that does not drain properly) and improperly tuned automation. Often, fish passages operate in cycles, with time settings (too long) and the number of repetitions (too few) not matching well with what works for migratory fish. Fish also cannot orientate themselves properly in the passages. In some cases, the attraction flow is too strong or too turbulent, or it is in the wrong place. In salt water, a fresh-water attraction flow sometimes rises vertically upwards, with fish unwilling to make the dive downwards. Finally, there are regularly technical problems and breakdowns. A notable incident was the occurrence of supersaturation of the water with oxygen, due to a pump sucking in air. In the process, fish formed air bubbles in their tissues.
Ideally, a fish passage should not only respect the fish's tolerance limits; the design should also seamlessly match instinctive behaviour. Knowledge of this is indispensable when evaluating fish passages and in drawing up the design. This will allow water managers to make a big impact in increasing eel and other migratory fish populations in the coming years! Dozens of pumping stations and locks along the Dutch coast are equipped with fish passages to allow migratory fish to travel inland. With the 'Fish Passage Check', it is possible to examine very precisely how fish passages function and whether improvement is possible, specifically for glass eels and other 'weak swimmers'. For example, research at the fish passage at the Schoute Pumping Station (Scheveningen) showed that, of the 125,000 glass eels that arrived in 2021, almost 30 per cent reached fresh water. This can go up to 75 per cent if the passage opens eight times a night, if the passage can continue to operate throughout the inland migration season and if the intake of the attraction flow is adjusted. Improvements were also found to be possible at other fish passages, in terms of both design and fine-tuning.
Thanks to the volunteers of Team Scheveningen (Samen voor de Aal) and to Wageningen Marine Research, Visserijbedrijf W.J. den Boer and Visserijservice Nederland.
Martijn Schiphouwer
(RAVON)
Maurice Kooiman
(RAVON)
Sanne Ploegaert
(RAVON)
Peter Jol
(Delfland Water Board)
Wil van der Ende
(Delfland Water Board)
Schoute pumping station from the outside (the seaside). In the middle the lock chamber c.q. fish passage. In the foreground the detector (left) and collector (right). Photo Martijn Schiphouwer
Summary
Griffioen, A.B. et al. (2018). Aalonderzoeken Hoogheemraadschap van Delfland: efficiëntie van glasaalintrek bij gemaal Schoute (Delfland Water Board eel surveys: Efficiency of glass eel inland migration at the Schoute Pumping Station). Report C007/18. Wageningen Marine Research (WUR)/RAVON Nijmegen.
Schiphouwer, M. et al. (2022). Landinwaartse migratie van glasaal via Scheveningen. Passagecheck complex Keersluis en Gemaal Schoute (Inland migration of glass eels via Scheveningen: Passage check of the Keersluis lock and Schoute Pumping Station complex). Project number 2020.053. RAVON, Nijmegen.
Kooiman, M. & S.M.A., Ploegaert (2020). Samen voor de Aal; Kruisnetmonitoring Zuid-Holland 2015-2020 (Together for the Eel; Cross-net monitoring in South Holland 2015–2020). Project number 2019.051. RAVON Foundation, Nijmegen.
^ Back to start
Focus on effectiveness
PFAS are a large and diverse group of fluorinated organic compounds. They are widely used: in numerous products, from clothing and non-stick pans to mobile phones, but also in the aviation and aerospace industries. PFAS barely degrade ('forever chemicals'), accumulate in the food chain and are even found in human blood[1]. They are associated with various health problems. It is estimated that at least 17,000 sites in Europe are contaminated with PFAS[2].
Many PFAS are readily soluble in water and difficult to degrade, or they break down into soluble and difficult-to-degrade compounds. PFAS enter the environment through the air, wastewater and waste. Because removal in sewage treatment plants and drinking water production is not always effective[3], they easily spread through the water chain. Small PFAS with short (C4–C6) and ultra-short (C2–C3) carbon chains in particular are very difficult to remove[4]. People in the Netherlands are exposed mainly through food, but also, albeit in low concentrations, through drinking water[5].
At 18 drinking water production sites in the Netherlands, both the source (11 surface water; 7 groundwater) and the drinking water produced were studied. Besides conventional techniques such as flocculation and aeration, advanced techniques such as activated carbon and ozone or UV light plus hydrogen peroxide are also used at some sites.
All surveyed sites are exposed to contamination from human activities. For surface waters, these include agriculture or wastewater treatment plants, for example. Human activities affecting groundwater sources may include local industrial activities (historical or otherwise), agriculture, infiltrating surface water and historical landfills. Moreover, they are not or hardly protected by hard-to-penetrate layers of clay. Therefore, the 7 selected groundwater sites are not representative of the 187 Dutch groundwater sources.
The 11 sites that use surface water are representative, though, namely for surface water in densely populated and highly industrialised deltas such as the western Netherlands.
The water samples were extracted at the University of Amsterdam and analysed using a newly developed method. This allows the measurement of many different PFAS compounds at very low concentrations[4].
In this study, we distinguish three groups of PFAS (see table):
• 20-PFAS, 20 PFAS from the Drinking Water Directive;
• 4-PFAS, four substances for which the European Food and Safety Authority (EFSA) has derived a non-binding opinion for tolerable weekly intake. Much is known about these four, including in terms of toxicology. They also belong to the 20-PFAS group and are the most important ones in it (PFOA and PFOS, among others);
• ultra-short PFAS, four substances with carbon chains of two or three carbon atoms. These substances fall outside the other two categories.
The graphs show the combined concentrations of the three PFAS groups in drinking water and drinking water sources. Note that the y-axes have a logarithmic scale to visualise the large concentration differences.
Although they are vulnerable extractions, groundwater sites score better in terms of PFAS than surface water sites. The combined concentrations of the EFSA 4-PFAS and the 20-PFAS from the European Drinking Water Directive are significantly higher in raw and purified drinking water from surface water (p < 0.001).
Ultra-short PFAS dominate in groundwater and surface water from the water sources studied: concentrations are a factor of 10 to 100 higher than for the other PFAS groups. Interestingly, concentrations in groundwater and surface water do not differ.
Trifluoroacetic acid (TFA), the smallest and most water-soluble PFAS, is the most common, with levels ranging from 90 to 521 nanograms per litre in groundwater sources and 82 to 641 nanograms per litre in surface water sources. TFA thus covers 76 per cent of measured PFAS in surface water and associated drinking water on average and as much as 95 per cent in groundwater and drinking water produced from groundwater. Thus, when this study discusses ultra-short PFAS, this mainly concerns TFA.
No natural sources of TFA are known[6]. TFA can enter surface water through atmospheric deposition. It can also enter groundwater, after leaching. In addition, TFA is a final degradation product of many PFAS substances that are partially degradable, including medicines, polymers, biocides and plant protection products.
The graphs also show concentration levels before and after purification (green and blue, respectively). Removal averaged 66 per cent for the EFSA's 4-PFAS and was moreover significant (p< 0.05). However, for the sum of the 20 PFAS (p= 0.17) and the ultra-short PFAS (p= 0.25), the removal is not significant. It was previously found that PFAS with shorter carbon chains are more difficult to remove from water[7]. The removal efficiencies differed between production sites, even when the sites used the same treatment techniques.
Human exposure to PFAS through food is highest. A smaller proportion passes through drinking water[5]. Nevertheless, the presence of PFAS in drinking water and sources of drinking water raises questions about emission pathways, human exposure and potential effects. The standards for PFAS in drinking water are regularly updated due to advancing knowledge. Limit values in the legally binding Drinking Water Directive (EU Drinking Water Directive) are considerably less stringent than the values for the 4-PFAS derived from the EFSA opinion.
The Drinking Water Directive has two criteria. The first sets a limit of up to 100 nanograms per litre for the total of 20 selected PFAS (20-PFAS); the other sets a limit of 500 nanograms per litre for all PFAS (total-PFAS). Both criteria are allowed by the EU. We have chosen the 20-PFAS here because it is impossible to measure all PFAS, and it is not clear which PFAS are included. According to the OECD (Organisation for Economic Co-operation and Development), there are 4,730 PFAS, while the US Environmental Protection Agency has 14,735 PFAS in its toxicity database, and the Open Chemistry database (PubChem) states that there are theoretically more than six million PFAS.
In our study, we did not find any instances of the sum standard for the 20-PFAS in drinking water being exceeded. However, 9 of the 11 drinking water samples from surface water did exceed the threshold derived from the EFSA opinion for the 4-PFAS (which is stricter but not binding), as also previously found by RIVM[5]. In summary, the drinking water produced from the groundwater sources studied complies with the binding directive as well as the EFSA opinion. However, drinking water from surface water does not always comply with the EFSA opinion. Drinking water companies are already making efforts to rectify this.
Despite these positive results, PFAS contaminants in groundwater are still a major challenge. This is because of the environmental behaviour of these substances and because remediation of PFAS-contaminated groundwater is very complex. For drinking water from ground and surface water, vigilance is key. Our results show the importance of considering a wide range of PFAS in water quality assessment and management. In addition, reducing the production and emission of PFAS is clearly important. This will protect both sources of drinking water and the wider environment and prevent PFAS from eventually getting into our drinking water as well as our food.
A European restriction proposal for all PFAS has been published, coming from five European countries including the Netherlands[8]. The earlier 2008 ban on PFOS (for many uses) resulted in lower concentrations in major rivers. A restriction therefore has a positive impact on surface water quality in the medium term. However, PFOS is still found in river water. This article deals with PFAS in surface water and groundwater, and in drinking water produced from these water sources (at 18 production sites in the Netherlands). The sum standard for 20 PFAS from the Drinking Water Directive was not exceeded anywhere. However, most drinking water samples from surface water did exceed the (stricter) limit value for four common PFAS recommended by the European Food and Safety Authority. The ultra-short PFAS are a particular point for concern. The existing standards and recommendations do not address these substances, even though they are by far the most common, especially trifluoroacetic acid (TFA). It is important to monitor PFAS in the air, soil, food and drinking water sources and to reduce emissions. Improved water treatment is also needed to reduce human exposure (drinking water treatment) and emissions (wastewater treatment).
How a restriction will affect concentrations in groundwater depends very much on the situation. Groundwater usually stays in the ground for a long time before it is extracted to produce drinking water. Moreover, PFAS are also still partially bound to soil particles or present in historical soil contamination. This means a restriction will only have a positive impact on groundwater quality in the much longer term.
Therefore, by limiting production and use now, we can avoid a bigger problem in the future. Meanwhile, it is important to continue monitoring emissions and levels of PFAS in the air, soil, food and drinking water sources. Improvement of water treatment techniques is also necessary to reduce emissions (wastewater treatment) and human exposure (drinking water treatment).
Mohammad Sadia
(University of Amsterdam)
Annemarie P. van Wezel
(University of Amsterdam)
Thomas L. ter Laak
(University of Amsterdam and KWR Water)
RIWA-Rijn in Nieuwegein; this is where river water is brought in for drinking water production. RIWA-Rhine photo
Summary
1. Göckener B. et al. (2020). Human biomonitoring of per- and polyfluoroalkyl substances in German blood plasma samples from 1982 to 2019, Environment International 2020: 145.
2. Dagorn G. et al. (2023). 'Forever pollution': Explore the map of Europe PFAS contamination, Paris, France: Le Monde; 2023, p. 7.
3. Eschauzier C. et al. (2011). Impact of Treatment Processes on the Removal of Perfluoroalkyl Acids from the Drinking Water Production Chain, Environmental Science & Technology 2011.
4. Sadia M. et al. (2023). Occurrence, Fate, and Related Health Risks of PFAS in Raw and Produced Drinking Water, Environ. Sci. Technol.
5. House of Representatives (2021). Letter from the Minister for the Environment, the Minister of Health and the Minister of Infrastructure and Water Management, kst-28089-190, https://zoek.officielebekendmakingen.nl/kst-28089-190.pdf; Published 4 June 2021.
6. Freeling F. & Björnsdotter M. K. (2023). Assessing the environmental occurrence of the anthropogenic contaminant trifluoroacetic acid (TFA), Current Opinion in Green and Sustainable Chemistry 2023: 41: 100807.
7. Rahman, M.F. et al. (2014). Behaviour and fate of perfluoroalkyl and polyfluoroalkyl substances (PFASs) in drinking water treatment: A review. Water Research 50, 318-340.
8. ECHA (2023). PFAS restriction proposal. ECHA/NR/23/04. https://echa.europa.eu/-/echa-publishes-pfas-restriction-proposal.
^ Back to start
in drinking water and water sources
Storm surge barriers are rarely used, but they should always work. The Maeslant Barrier in the Nieuwe Waterweg canal closed automatically for the first time during the storm surge on 21 December 2023, 26 years after its commissioning. Until then, it had only experienced test closures and closures due to the manager’s intervention. How do we ensure the necessary knowledge and skills to keep the barrier in working condition? Rijkswaterstaat is exploring the possibilities of a simulator, also known as a digital twin.
The Maeslant Barrier consists of two huge gates on the banks in a kind of dry dock. When the barrier is closed, the gates move towards each other, floating on the water. The gates, which now form a retaining wall, fill with water and sink to land on a sill structure at the bottom. When the barrier reopens, the gates are pumped empty and move back into the dry dock. A computer system 'decides' whether to close, and closure also happens automatically, with operating personnel as back-up.
To keep the Maeslant Barrier operational, a strict risk-based asset management method called ProBo ('Probabilistic Operations and Maintenance') is applied. This is very knowledge-intensive, since the barrier is unique, complex and rarely used. Like other organisations, Rijkswaterstaat is faced with the challenge of attracting and retaining young technical workers for a longer period of time. That gets in the way of the structural transfer of knowledge. While the safety of flood defences is ensured, opportunities to improve operations and maintenance may be missed. Digital twins have proven their worth in aviation, aerospace, manufacturing and offshore industries, for example. A study into its application for storm surge barriers was therefore a logical next step.
A digital twin is a digital replica of a physical system captured in a software model. From a user interface (often a 3D model or GIS environment), data are visualised, the physical object can be monitored and simulations are possible. So a digital twin not only contains visualisation capabilities but is also supported by computational models that allow a broader interpretation of the data in the background. Based on this, actions may follow (by human hands or automated) to modify the physical object. It is a promising technology, but it has yet to see much use in the water safety sector. Meanwhile, several Proof of Concept studies have already demonstrated its potential.
Creating a prototype of a digital twin of the Maeslant Barrier required integrating knowledge from three areas: 1) organisational and user needs; 2) barrier operation (civil, mechanical and electrical engineering); and 3) IT architecture design.
Four applications where a digital twin can add value:
1. improved efficiency of knowledge and information management;
2. cost savings resulting from better monitoring of the barrier;
3. better risk management using data driven models and analysis;
4. providing insight into the behaviour of the barrier during closure.
Based on design requirements from a preliminary study, we built a digital twin prototype. To do so, we used Unity for the visual 3D animation and Python scripts for data calibration and linking external models. Those external models are:
• a hydrostatic force model;
• a closure reliability model;
• a pump performance model.
The Unity environment functions as a user interface integrating a 3D data animation model with data visualisation and data analysis panels. This prototype makes it possible to recreate the closure of the barrier in 3D based on measured or simulated data.
The focus in the prototype is on the Maeslant Barrier's retaining wall. This led to the decision not to process all measured parameters of a closure (6,400 in total). In particular, we are currently limiting ourselves to water levels on the Nieuwe Waterweg canal, water levels in the 13 compartments of the retaining wall, pumping capacities of the approximately 35 pumps (per wall) and the position of the retaining wall (horizontal, vertical and rotation).
The linked models and automated data analysis provide instant insight into the state of related components. This allows for more efficient asset management of the barrier. As an example, a failing pump can be predicted based on pump power versus flow rate measurements. Next, the impact of the failing pump on the barrier as a whole is analysed directly. If it loses little in terms of reliability, repair is possible, and quick (and expensive) replacement is not necessary.
The coupled hydrostatic force model gives an impression of the forces acting during the closing process. This is obviously not yet complete, but it can already clarify the basic principles of the forces to new employees. In future versions, it will be possible to make this more and more detailed, which is highly desirable in order to get an accurate picture of the forces acting on the barrier.
The prototype was tested among a wide group of potential users within Rijkswaterstaat. This quickly showed that the digital twin can indeed play a key role in knowledge storage and transfer, for example when it comes to force generation. It is important to be able to look back on closures, how they took place and what maintenance and replacements were done. This is necessary to be able to look ahead and avoid repeating incidents. With the prototype, it is already possible to simulate the closure process of the barrier over and over again based on measurements. By replaying these digitally, the behaviour of the barrier (e.g. the skewed angle of the retaining wall or the performance of components) in different high-water situations can be analysed. Deviations in digital or other behaviour are observed more quickly, and protocols can be adjusted if necessary.
The testers found the digital twin promising for the overall asset management of the barrier, from automating data analysis for maintenance to simulating 'what if' scenarios. The capabilities are limited at the moment, but in subsequent versions, expansion of these functionalities is certainly possible.
It appears possible to build a large-scale digital twin for the Maeslant Barrier with the hardware and software currently available on the market. To run a true, full digital twin, hardware and software improvements are still needed within Rijkswaterstaat, especially in the area of cyber security.
Although testers are broadly positive, executive engineers in particular are still somewhat hesitant. However, most of the Maeslant Barrier's asset management organisation is enthusiastic. Moreover, a large-scale digital twin also appears to be financially viable: an indicative business case indicates that a digital twin will be cost-effective within five years. Further development of the prototype is therefore the obvious choice, in which regard a good connection to user needs (the executive technicians!) will require a lot of attention.
Therefore, the biggest challenge for implementation is not so much the technology but getting the organisation on board. A digital twin implies a new 'way of working', which often leads to resistance at first. However, by putting the user perspective first and continuing to work in small steps, progress is certainly possible.
Digital twins do not necessarily provide a perfect solution to the challenges for Rijkswaterstaat around knowledge management and storm surge barrier management. They can certainly help, as the barriers themselves produce enough data to feed digital twins and keep improving. Rijkswaterstaat, TU Delft and Aveco de Bondt developed a prototype digital twin for the Maeslant Barrier. The prototype integrates a 3D animation model with data visualisation panels and simulation models. The prototype has functional applications, such as analysis of closures (forces, pump operation), knowledge transfer and improving management (including risk management) and maintenance. The prototype has been tested among users, who recognise the added value for knowledge and information management and also for improving management and maintenance. A large-scale digital twin for the Maeslant Barrier appears to be feasible, despite challenges such as cyber security. Large-scale implementation will require further development and better alignment with user needs of technicians and future users.
The research over the past two years provides a good basis for further development. Those involved see clear added value, and digital twins are technically, financially and organisationally feasible. It is now up to Rijkswaterstaat to take this further.
This knowledge is valuable not only for the Netherlands but also elsewhere in the world. Digital twins can be included as early as the design phase to strengthen management and maintenance of flood defences. This could be applied, for example, in the new storm surge barrier being developed near Houston, Texas, for the US Army Corps of Engineers.
When using the digital twin, you work from a 3D model of the barrier. When running a scenario, you see the barrier move based on measured or simulated data. Forces at play are indicated through arrows, and the retaining wall can be made transparent to see how the retaining walls fill with water. You can also see how the arms rotate during the sinking of the retaining wall. Additional information can be requested during the simulation, for example in the form of data visualisation, documentation or videos.
Luc Ponsioen
(Aveco de Bondt/ TU Delft)
Bas Jonkman
(TU Delft)
Alexander Bakker
(Rijkswaterstaat)
The Maeslant Barrier at Hoek van Holland. Rijkswaterstaat photo
Summary
Ponsioen, L.A. et al. (2023). Investigation and development of a Digital Twin for the Maeslant barrier: Exploring the application of digital twins in the maintenance and operation of storm surge barriers. EngD research report. http://resolver.tudelft.nl/uuid:532df076-927d-4a07-8114-def359198c90
^ Back to start
Case study Maeslant Barrier
In summer, blue-green algae grow in many Dutch lakes. Water managers can issue a warning or a negative bathing advice based on periodic sampling. However, the amount of blue-green algae varies greatly depending on the time and place, partly due to weather conditions. As a result, despite a warning or negative advice, the water in between sampling times may still be safe, or unsafe despite no warning or negative advice having been issued. Can the Algae Radar help make bathing advice more reliable?
The current practice is that of the Blue-Green Algae Protocol 2020[1]. This protocol provides guidelines for drawing up bathing advice: the water manager measures blue-green algae concentrations on a weekly or bi-weekly basis and bases bathing advice on these: warning (12–75 µg/L), negative bathing advice (>75 µg/L). The problem with this method is that the data are never up to date. It would be better if blue-green algae concentrations could be predicted. To that end, the Algae Radar is being developed (see box). The Algae Radar links periodic measurements of blue-green algae to historical average weather data from previous years.
The Algae Radar is still under development. It is a data-driven model from Deltares that produces blue-green algae forecasts based on blue-green algae measurements and meteorological data (wind speed, air temperature and solar radiation[2]. Previously, Algae Radar forecasts were made exclusively based on weather averages from previous years. This project uses current weather forecasts for the first time. In this way, the blue-green algae forecast is given a level of uncertainty linked to the uncertainty in the weather forecast – the blue-green algae plume. The expectations visualise the extent to which risk levels (warning or negative bathing advice) are exceeded (Figure 1).
Sometimes, blue-green algae near the banks can cause additional nuisance by forming floating layers. The latter results from the movement of water and leads to extremely high concentrations. This phenomenon cannot be predicted by the Algae Radar, since water movement is not included in the model. However, it is possible to take measures, such as flushing or peroxide treatment, based on the Algae Radar forecast, even before the nuisance starts. The Algae Radar can also be used to test the effectiveness of measures.
To this end, we conducted high-frequency measurements of blue-green algae and other relevant parameters in two Dutch lakes during the bathing season from 1 May to 1 October 2022: the Paterswoldsemeer lake near Groningen and the Wylerbergmeer lake near Nijmegen. High-frequency measurements in Paterswoldsemeer were compared with sampling by the Noorderzijlvest Water Board and the Algae Radar blue-green algae forecasting model.
For the high-frequency measurements in this project, NIOO-KNAW is using two new measuring buoys. These determine concentrations of blue-green algae and total chlorophyll every 10 minutes at a depth of 50 centimetres based on measurements of fluorescence, oxygen, acidity and conductivity. The buoys are powered by a solar panel and send hourly data to a server, from where the data can be analysed. They thus provide a near real-time picture of the various parameters and thus insight into the daily dynamics of blue-green algae.
The KNMI has access to ensemble weather forecasts of temperatures and other meteorological quantities – the 'weather plume'[3]. These are 51 separate forecasts, which together give a good idea of the possible weather over the next two weeks. The ensemble weather forecast thus gives the uncertainty of that forecast in addition to the forecast itself. The ensemble weather forecast and blue-green algae measurements (standardised according to the Blue-Green Algae Protocol) are entered into the Algae Radar.
The buoy measurements show daily dynamics between mid-August and mid-September 2022 (Figure 1). Immediately after the start of measurements, the blue-green algae concentration increased for a period of about one week, leading to a negative bathing advice (Figure 1A, B). After this period, concentrations decreased and, based on the buoy measurements, the advice would change to a warning around the beginning of September. The Algae Radar's blue-green algae forecast model showed a similar pattern based on the five-day weather forecast, but now the concentration remained high until just before the end of the measured period, resulting in a negative bathing advice for almost the entire period. The buoy measurements also show some variation throughout the day, where in some cases the lowest measured values would arrive at a different advice than if the average were used.
The results from the Algae Radar model remained above the level of a negative bathing advice for almost the entire period from 15 August to 1 September. As mentioned earlier, the Algae Radar calculates blue-green algae concentrations based on meteorology and blue-green algae measurements. For the latter, the water board's monthly measurements were used. The most recent measurements weigh most heavily in determining future concentrations. Figure 1D shows that the mid-August reading is high (grey bar). Combined with weather conditions favourable to blue-green algae (high temperature, high irradiance and low wind speeds), this leads to model results remaining high throughout the period until mid-September. After all, the model adjusts expectations only once new blue-green algae measurement data come in, and they did not come in during this period. In the future, it will be possible to use data from the measurement buoys. However, this will not be possible for another few years, because calibrating the Algae Radar has so far required a historical measurement series of at least six years. This period can be shortened by measuring more frequently with the measurement buoy.
The buoy measurements show that the concentration of blue-green algae can vary more than is visible with less frequent field sampling. In this case, this leads to an overestimation of the risk in the second half of the measurement period. The Blue-Green Algae Protocol 2020 prescribes weekly or bi-weekly measurements. If followed, it is plausible that, for the second period as well, the advice issued would better reflect the buoy measurements and thus the actual situation. Therefore, for the limited dynamics during such a short measurement period, standard monitoring will be sufficient. It is possible that daily dynamics will increase during the initial phase of blue-green algae blooms and/or stronger variation in weather. In that case, buoy measurements can help by providing a remote, direct view of the situation at a bathing site. In addition, targeted monitoring based on such buoy measurements could even be possible, with the possibility to take additional field measurements any time the buoy changes its advice.
Climate change is causing temperatures to rise. This is also expected to increase nuisance from blue-green algae. As a result, bathing sites may be closed more often and for longer. At the same time, there is a greater need to cool down. Water managers can use water forecasts to more accurately, and in advance, determine the risk of standards being exceeded. This will allow more effective and risk-driven sampling, i.e. with less cost and effort, allow any measures to be taken earlier and potentially allow bathing sites to remain open more often.
If the water forecast is to be used in practice, the buoy measurements could be integrated into the forecast model to increase short-term certainty. In addition, the reliability of the forecast can be tested for different conditions, such as the depth and size of a body of water or the amount of blue-green algae. Finally, a dashboard that directly displays measured data and model forecasts would be useful. This will allow us to view blue-green algae concentrations almost in real time, as well as the forecast for the coming days. As a result, targeted monitoring will be possible, for example by not sampling if the situation is expected to remain unchanged. For an even better expectation of risks, the expectation model should be extended to include toxin concentrations. After all, the health risk is due to the toxins excreted by the blue-green algae[4].
This project was funded from the Digital Government 2021 Innovation Budget under the name Voorspellen Veilig Zwemwater (Predicting Safe Bathing Water), project number 1500025049091. The project was carried out under the supervision of stakeholders from Leisurelands, the Rivierenland Water Board, the Noorderzijlvest Water Board and the Dutch Association of Regional Water Authorities. During blue-green algae blooms in summer, bathing water managers may issue a warning or negative bathing advice. However, the amount of blue-green algae varies greatly depending on the time and place, partly due to weather conditions. As a result, the bathing advice may temporarily not match the actual water quality in between sampling sessions. Based on an expectation of blue-green algae, sampling can be risk-driven and bathing waters can reopen earlier after nuisance. This project has taken a first step for such an expectation. During the 2022 bathing season, high-frequency measurements of blue-green algae and other relevant parameters were taken in two lakes in the Netherlands. The measurements were compared with a forecast using the Algae Radar model – a 'water forecast' – based on low-frequency measurements by the water board and detailed weather forecasts.
Miguel Dionisio Pires
(Deltares; Aeres University of Applied Sciences Almere)
Peter Siegmund
(Royal Netherlands Meteorological Institute)
Sven Teurlincx
(Netherlands Institute of Ecology)
Dedmer van de Waal
(Netherlands Institute of Ecology; University of Amsterdam)
Marc Weeber
(Deltares)
The Water Forecast: information board at the Wylerbergmeer lake
Summary
1. Sketch, F.M., et al. (2020), Blauwalgenprotocol 2020 (Blue-Green Algae Protocol 2020), RIVM letter report 2020-0107.
2. Deltares: Algenradar voorspelt algenbloei (Algae Radar predicts algal blooms). https://cms.deltares.nl/assets/common/downloads/Flyer-Algenradar-voorspelt-algenbloei-1.pdf
3. KNMI: Weer- en klimaatpluim en Expertpluim (Weather and climate plume and Expert plume), https://www.knmi.nl/nederland-nu/weer/waarschuwingen-en-verwachtingen/weer-en-klimaatpluim
4. Van de Waal, D.B., et al. (2023), Risicobeoordeling van blauwalgen: snel, nauwkeurig of beide? (Blue-green algae risk assessment: Fast, accurate or both?). Water Matters no. 17, December 2023.
^ Back to start
Algae radar predicts
The Dutch canals beautify the cityscape and offer residents of cities such as Amsterdam, Utrecht and Groningen entertainment and cooling on hot days. However, not all of those urban water bodies are all that clean. Some canals become overgrown with algae or duckweed, while others are highly turbid due to upturned sediment. Sure, the canals are cleaner than they were a century ago, but there is still much to improve, in terms of both ecology and the climate.
The Netherlands has between 100–250 km2 of urban water bodies[1] . This includes Amsterdam's canals, but also small ditches, streams and ponds in residential areas. This means an urban water body does not necessarily refer to a canal with a brick embankment – it can also have a grass bank in a city park. It is important to understand what factors play a role in the ecological functioning of canals. Greenhouse gas emissions from urban water bodies are a big unknown. Agricultural drainage ditches and shallow lakes in the Netherlands are known to be sources of the greenhouse gases carbon dioxide (CO2) and methane (CH4). With regard to urban water bodies, we know almost nothing about this.
Greenhouse gas emissions from water are the result of microbial processes. In a balanced ecosystem, algae and aquatic plants take up nutrients (such as nitrate and phosphate) and use them to grow. In the process, they also sequester CO2. When the biomass dies, it is broken down by bacteria in the water and in the upper sediment layer. This requires oxygen. The bacteria convert the biomass into new bacteria (reproduction), and CO2 is released again. The deeper part of the biomass remains in the soil and, in that low-oxygen environment, is slowly decomposed into methane. In the upper layers of the sediment, this methane can be converted back to CO2 by bacteria that 'eat' methane: methanotrophs. If this balance is disturbed by warming or excessive nutrient addition (i.e. lots of biomass), the balance shifts to a system with less oxygen but higher oxygen demand. A higher proportion of biomass is then converted to CH4. The excess CH4 escapes mainly through bubbles, giving the methanotrophs no chance to convert it to CO2, resulting in methane entering the atmosphere. Although it has a shorter half-life than CO2, it is also 27 times stronger as a greenhouse gas than CO2[2] .
Although this may not be something people think about a lot, microbes are very important for biodiversity. They break down much of the organic matter, or remove nitrogen compounds by converting them into nitrogen gas. Microbiological activity thus helps determine water quality and greenhouse gas emissions.
To get a better picture of microbiological activity in urban water bodies, canals in six Dutch cities were figuratively put under the microscope. Nutrient concentrations in surface water were measured in the lab and in the field, and the potential CH4 emissions were determined.
In several fieldwork campaigns between 2019 and 2022, diffuse emissions of CH4 and CO2 were measured with floating chambers on the surface of the water (Figures 1 and 2). What is immediately noticeable is the wide variations between cities. Phosphate concentrations and CO2 fluxes are highest in Den Helder and Zaandam. Conversely, in Amsterdam and Middelburg, CO2 was actually being removed from the atmosphere by algae in the water. This can be explained by the sunny conditions during the measurements and the season (spring). The differences in phosphate, nitrate and ammonium concentrations are striking but difficult to explain. All measurements were taken between March and July in the city centres. Nutrient discharge at a sewer overflow could explain the phosphate, but this is not very likely, as there was no heavy rainfall during the measurement days.
Methane emissions appear limited (except for an outlier in Zwolle) but converted to CO2 equivalents are almost as high as the average measured CO2 emissions (1 g/m2/d). Moreover, CO2 can readily be sequestered by plants and can remain dissolved as bicarbonate. CH4, on the other hand, is poorly soluble in water, making gas bubbles a major source of emissions. These were not measured in this study, so it is plausible that actual emissions are higher than what we currently see in the diffusive flux measurements.
A typical feature of canals and other urban water bodies is the canal walls. In city ponds and ditches, the walls consist of wooden planks; in the inner city, they are mainly made of brick. A slime layer (biofilm) also known as periphyton forms on the canal walls when water levels vary little. This is a complex ecosystem of mosses, cyanobacteria, heterotrophic bacteria and protists. DNA analysis of all bacteria on the canal wall found methanotrophic bacteria, among others[1]. Four cities turned out to have as similar community of bacteria. In Zwolle, at one site, as much as 9% of all bacterial species on the canal walls were methanotrophic.
On the canal walls in Leiden and Middelburg, things were different. Leiden's canals, such as the Rapenburg and Oude Vest canals, were characterised by high visibility and aquatic plants on the bed of the canal. What is striking is that phosphate and ammonium concentrations were low here as well, as were methane emissions. In Middelburg, most of the water is in direct connection with the Western Scheldt estuary. The water is therefore salty (around 20 ppt), which affects the carbon cycle. As a result, salt water generally has lower methane emissions than fresh water[3]. CO2 uptake from the air indicates active photosynthesis, and the many algae in the water provided visual confirmation of this. Whether urban water bodies in Middelburg will emit a lot of CH4 despite the influence of the North Sea when the algae die off is a relevant question for follow-up research.
With rising global temperatures and a greater need for open water in the city, canals will not be disappearing from the typical Dutch urban landscape any time soon. A future-proof urban water policy starts with understanding the processes that affect our living environment. Greenhouse gas emissions are merely one piece of the puzzle. This research makes it clear that human impact on urban water bodies has not only increased nutrient concentrations but also contributes to the carbon footprint. A holistic view of the approach to urban water quality is crucial. The ecological state of urban water bodies is under pressure due to increased nutrients and climate change. This may be accompanied by increased emissions of methane and carbon dioxide. In six Dutch cities, greenhouse gas emissions were measured in the spring and the microbiological composition was determined. Almost all canals emit methane, while carbon dioxide is sometimes absorbed. Canals with more biodiversity emit less greenhouse gases. Canal walls can also act as a habitat for methanotrophic bacteria. Improving urban water quality will not only improve biodiversity but also the impact of urban water bodies on the climate.
Urban water bodies are environments created by humans, which we can influence ourselves. This means several possibilities are available to us. For example, we can adjust water levels and flow rates and address nutrient sources. An improvement in turbidity was measured during the coronavirus pandemic thanks to the boating ban in the Amsterdam city centre[4]. Soon, aquatic plants were given a chance, showing that rapid improvement in water quality is possible.
Run-off of nutrients from the street to surface water following rainfall cannot be completely prevented, but a discharge from the sewer often can be.
Periodically adjusting the flow in an inner city can help prevent blue-green and other algae blooms, reduce greenhouse gas emissions and give aquatic plants a chance. More aquatic plants and fewer ships is also beneficial for fish and aquatic insects. Moreover, aquatic plants not only affect the water but also the soil. Through the roots, plants can excrete oxygen, reducing CH4 emissions.
An immediate recommendation, therefore, is to artificially increase the flow of water within cities. This is a fairly simple measure that can have many positive effects.
To make better trade-offs in terms of greenhouse gas emissions, knowledge about annual distribution and emissions via bubbles is needed. For water quality, it is also important to identify where in the urban water network the sources of nutrients lie.
Municipalities paying attention to managing their urban water bodies can kill two birds with one stone: a more pleasant urban environment through higher water quality and biodiversity, and lower greenhouse gas emissions.
With thanks to Cornelia Welte and Mike Jetten (Radboud University) and Joshua Dean (University of Bristol).
Koen Pelsma
(Deltares)
Wouter van der Star
(Deltares)
Bloemgracht in Amsterdam, 2019
Summary
1. Pelsma, K. A. J. (2023). Hidden beneath the surface: microbial methane cycling in Dutch urban canals (Doctoral dissertation, Radboud University). https://hdl.handle.net/2066/299121
2. Forster, P. et al. (2021). The Earth's Energy Budget, Climate Feedbacks, and Climate Sensitivity. In Climate Change 2021: The Physical Science Basis. Contribution of Working Group I to the Sixth Assessment Report of the Intergovernmental Panel on Climate Change. Cambridge University Press, Cambridge, United Kingdom and New York, NY, USA, pp. 923–1054, https://doi.org/10.1017/9781009157896.009
3. Saunois, M. et al. (2019). The global methane budget 2000–2017. Earth System Science Data Discussions, 2019, 1-136. https://doi.org/10.5194/essd-12-1561-2020 H2O Actueel (May 28, 2020). Amsterdamse grachten helderder dan ooit dankzij coronamaatregelen (Amsterdam canals clearer than ever thanks to coronavirus measures) https://www.h2owaternetwerk.nl/h2o-actueel/amsterdamse-grachten-helderder-dan-ooit-dankzij-coronamaatregelen.
^ Back to start
How to improve quality
Contaminated surface water is potentially a source of human infection with antimicrobial resistant (AMR) bacteria. It is known that these bacteria can enter surface water via effluent from wastewater treatment plants and from combined sewer overflows. To what extent do illicit connections in separate stormwater systems also contribute to this?
Infection with AMR bacteria (transmission) may occur when people come into contact with contaminated surface water, for example as recreational users. AMR bacteria can enter surface water in different ways. For example, they can do so through animal manure run-off from livestock farming or human wastewater discharge via effluent from wastewater treatment plants and combined sewer overflow. The role of discharges due to illicit connections in separate wastewater sewer systems is unclear. With more and more separated systems being introduced, clarity on this is becoming more important.
Due to illicit connections in separate wastewater sewer systems, e.g. house connections being swapped around, untreated wastewater can unintentionally enter surface water via the stormwater system. Illicit connections can thus be a source of faecal contamination carrying AMR bacteria. Previous research[1] has demonstrated the presence of AMR bacteria in surface water. As influences from agriculture, effluent from wastewater treatment plants and combined sewer overflows were absent, illicit connections were identified as the most likely source. The question is whether that is justified.
This article describes the results of a study into the relationship between illicit connections in separate stormwater systems and the presence of AMR and other bacteria in the receiving surface water. For this purpose, water quality was examined in a single area before and after the illicit connections were detected and repaired.
The 'Heuvel' research area is located in a residential area in the south-west of Breda. The area originally had a combined sewer system, but separate wastewater and stormwater sewer systems were recently installed. The surveyed stormwater system (total pipe length of about 4.5 km) is a gravity system that drains to one of nine outlets on a central pond. The outlets are fitted with non-return valves to prevent inflow from the pond into the system. The receiving surface water in the district (the Talmazone pond and the Turfvaart watercourse) is small and therefore sensitive to the effects of illicit connections. Further downstream in the Aa of Weerijs brook and in the centre of Breda, plans for recreation near and in the surface water exist, which makes good water quality extra important.
Based on inspection results and reports, there was a suspicion that there were one or more illicit connections in the stormwater system. Several illicit connections were indeed found in this investigation. They were detected using the distributed temperature sensing (DTS) technique: fibre-optic cables temporarily inserted in the sewers measure the temperature in the stormwater sewer. Illicit connections can be detected based on sudden temperature variations. Based on the discharge pattern observed in this way, the illicit connections were classified: multiple households with toilet, one household with toilet, other (without toilet). The locations and categories of the illicit connections found are shown in Figures 1 and 2.
For the faecal contamination survey, samples evenly distributed over the stormwater system (sites 1 to 23) and surface water (sites O1 to O3) were taken. The samples were analysed for E. coli and for ESBL-EC.
E. coli is the standard indicator bacterium for faecal contamination. The bacteria are found in the intestines and faeces of all warm-blooded animals, including humans.
ESBL-EC is an antibiotic resistant variant of E. coli. Such resistance can cause problems in treating infections. About 5% of all Dutch people are carriers of ESBL-EC. The resistant bacterium is also frequently found in dogs (about 11%) and waterfowl (about 16%). Furthermore, ESBL-EC is also regularly found in the intestines and manure of farm animals, especially in poultry, fattening pigs and veal calves[2,3,4,5].
The presence of E. coli or ESBL-EC in a water sample indicates the presence of faecal contamination. The origin of that contamination can be determined with DNA analysis. In this study, a gene (HF183) of the bacterial species Bacteroides dorei was used as an indicator for human faeces, a DNA fragment of an avian-specific Helicobacter for that of birds and canine mitochondrial DNA for canine faeces.
Samples were taken from the stormwater system and surface water in three rounds of three or four days each: two rounds (in June and November 2019) before illicit connections were repaired and one round (in September 2022) after repair. All water samples were examined for the presence of human faeces. Only the samples from June 2019 (i.e. before the repair of illicit connections) were additionally analysed for faeces from birds and from dogs. See Table 1.
Human-specific DNA was found in 44% of the samples taken before the illicit connections were repaired, especially close to the misconnection sites (see Figure 1, the top row of dots per site). After repair (bottom row of dots), human-specific DNA was only found near one illicit connection that had not yet been repaired (yellow dot near sites 2 and 3). The effect of remedying a large illicit connection (multiple households with toilets) is easily visible at site 8, and downstream towards sites 7 and 6: all samples contained high concentrations of Bacteroides before repair and were negative after repair.
During the sampling round in June 2019, canine DNA was found in 6 out of 15 samples from the stormwater system (40%); it is not found it in the surface water samples. Bird-specific DNA was found in only one surface water sample.
The blue arrows indicate the sampling location.
Figure 2 shows concentrations of E. coli and ESBL-EC for each site before repair of illicit connections (top row of dots) and after repair (bottom row). What is striking is that the concentrations of both did not decrease after the illicit connection was fixed. For ESBL-EC, the percentage of positive samples even increases from around 20% to around 80%.
The DNA analyses showed that the central part of the system contained almost no traces of human faeces after the repair of illicit connections. The fact that ESBL-EC is actually more widespread (and E. coli concentrations are not decreasing) in spite of this suggests that sources other than human wastewater are contributing to the presence of these bacteria. Dog faeces are a possible source of the antibiotic-resistant ESBL-EC. The presence of dog faeces in the stormwater system was not determined for the samples from September 2022 (after repair), but about half of the earlier samples from June 2019 did contain dog DNA. Three of the four samples that were positive for ESBL-EC in June 2019 were also positive for canine DNA.
The study shows that human-specific DNA was present in high concentrations before the illicit connections were repaired and virtually absent thereafter. This means the repairs prevented the discharge of human sewage into the stormwater system. Antimicrobial resistant (AMR) bacteria enter surface water through several routes. Besides wastewater treatment plants and combined sewer overflows, illicit connections in separate sewer systems are another potential source. A study of a small stormwater system with known illicit connections showed that repairing the illicit connections did lead to the (near) elimination of human wastewater in the system, but this was not accompanied by a sharp decrease in the amount of E. coli and the antibiotic resistant variant ESBL-EC. This means other sources should be considered, such as run-off of animal faeces (e.g. dog faeces), as possible sources of AMR and other bacteria.
E. coli and ESBL-EC were still, however, present in the samples in roughly equal proportions before and after the illicit connections were repaired. No clear decrease was found for these bacteria. Thus, the E. coli and ESBL-EC in the stormwater system probably did not originate exclusively from wastewater due to illicit connections. The source may be run-off of dog faeces into the stormwater system with the rain. Dogs are known carriers of ESBL-EC, and canine DNA has been detected in some samples from the stormwater system.
Thus, the presence of E.coli and ESBL in stormwater run-off does not necessarily indicate the presence of illicit connections. Supplementation with DNA analysis on human markers is needed. A point of attention in this type of study is conducting multi-day sampling campaigns to limit the influence of accidental outliers (as at site 10: one strong positive result for Bacteroides, with negative results on the other three days).
Fixing illicit connections will certainly reduce the emission of AMR and other bacteria from rainwater systems, but other sources, such as dog faeces run-off, may also play a role in the discharge of AMR and other bacteria to surface water.
Rémy Schilperoort
(P4UW)
Jeroen Langeveld
(P4UW)
Heike Schmitt
(RIVM)
Hetty Blaak
(RIVM)
Martijn Klootwijk
(Municipality of Breda)
Bert Palsma
(STOWA)
Sampling from a storm water drain, with behind it the bus that is tracing illicit connections
Summary
1. Schilperoort et al. (2017). Antibioticaresistente bacteriën in stedelijk water en de rol van foutaansluitingen (Antimicrobial resistant bacteria in urban water bodies and the role of illicit connections). H2O Water Matters journal, December 2017, 12-15
2. Van der Bunt et al. (2019). Prevalence, risk factors and genetic characterisation of extended-spectrum beta-lactamase and carbapenemase-producing Enterobacteriaceae (ESBL-E and CPE): a community-based cross-sectional study, the Netherlands, 2014 to 2016. Euro Surveill 24 (41). https://doi.org/10.2807/1560-7917.ES.2019.24.41.1800594
3. Van der Bunt et al. (2020). Faecal carriage, risk factors, acquisition and persistence of ESBL-producing Enterobacteriaceae in dogs and cats and co-carriage with humans belonging to the same household. J. Antimicrob Chemother 2020:75 (342-350). https://doi.org/10.1093/jac/dkz462
4. Veldman et al. (2013). Characteristics of Cefotaxime-Resistant Escherichia coli from Wild Birds in The Netherlands. Applied and Environmental Microbiology 79 (24), 7556-7561.
5. Mevius et al. (Ed.) (2018). ESBL-Attribution analysis (ESBLAT): Looking for sources of antibiotica resistance in humans. TKI Agri & Food.
^ Back to start
More spreading of ABR bacteria?
For years, the busy Bleiswijkse Zoom water area and greenbelt in the Rottemeren area of South Holland was plagued by cyanobacteria and the accompanying negative bathing advice. Also, Bleiswijkse Zoom did not meet the WFD objectives. To restore the ecosystem to a stable, clear condition with submerged aquatic plants, an integrated system approach was applied. What are the effects of the measures taken?
For years, Bleiswijkse Zoom, a recreational lake of over 14 hectares dug near Rotterdam around 1970, had a fixed water level. Water was brought in from the Rotte river to maintain the lake’s water level and to supply water to the area behind it for the purpose of maintaining the water level in this area. The supplied water was nutrient-rich, making the water in the lake hypertrophic. On top of a nutrient-rich layer of silt, the water was rich in algae and turbid. The banks were mostly steep and fitted with reinforcements, with little room for good riparian vegetation. The macrofauna in the lake consisted mainly of negative indicator species, and there was a high biomass of fish, mostly bottom-dwelling fish. Aquatic plants were almost entirely absent.
To gain insight into the water-quality problem in Bleiswijkse Zoom, the district water authority used a systems analysis[1] to investigate the Ecological Key Factors (EKF) [2]. This revealed that:
• the external phosphate load (total-P) was too high; it was above the critical limits at 7 milligrams P/m2/day. The critical limits being 5.0 mg P/m2/d for a 'transition from clear to turbid' and 3.0 mg P/m2/d for a 'transition from turbid to clear'. The main sources of phosphate were water from external sources to maintain the water level, seepage water, leaching and run-off;
• the light climate was inadequate. Transparency was limited by algae and suspended matter (due to bottom-dwelling fish);
• Continued supply of phosphate from the lake bed was high (3.5 mg P/m2/d)[3].
Reducing the external phosphate load to below the lower critical limit – for a transition from turbid to clear, plant-rich water – proved unfeasible. A combination of source, systemic and internal measures was needed to reduce the load to 'between critical limits'. In addition, to force a transition 'from turbid to clear', fish stocks were depleted and the continued supply of phosphate from the lake bed was reduced.
The water authority chose to focus first on measures aimed at reducing water productivity (EKF 1), then on improving the light climate (EKF 2) and finally on reducing sediment productivity (EKF 3). In short, the main focus has been on source measures that reduce the phosphate load and measures to enable a final transition to a clear state. The robustness of the system will be addressed by establishing a flexible water level and expanding the riparian zone with helophytes.
Measure 1: limiting water intake from the Rotte river
By constructing a bypass, Bleiswijkse Zoom no longer functions as an intermediary location to hold water. The nutrient-rich Rotte water now passes underneath the lake into the area behind it. In addition, the water requirements of Bleiswijkse Zoom have been greatly reduced by setting a flexible water level that can vary by as much as 55 centimetres. Rain now provides most of the replenishment, and water from the Rotte river is only used at very low water levels. The reduction of the intake to practically zero results in a decrease in the external phosphate load of almost 2 milligrams P/m2/d. Moreover, the large level margin is beneficial for vegetation: riparian vegetation is expanding naturally, and the shallower water in summer allows light to penetrate to the bottom more easily. Also, algae are no longer introduced via the water added to the lake to maintain the water level.
Measure 2: creation of additional riparian zones
The construction of more nature-friendly banks and natural expansion of existing reed banks (by removing reinforcements in combination with a flexible water level) has increased the purification capacity of the lake and has given the system greater ability to support life. This makes the lake more robust and raises the aforementioned critical limits.
Measure 3: reducing turbidity caused by fish
The rather large fish stock (423 kg/ha) was dominated by bottom-dwelling carp and bream[4]. This was detrimental for the light climate, but this fish stock also does not fit the new, nutrient-poor situation. Reduction was carried out, resulting in a maximum of 15 kg/ha of plankton-eating fish and 30 kg/ha of bottom-dwelling fish[5]. Some large carp have been retained for angling purposes. Excess fish were moved – in consultation with the angling federation – to suitable angling waters.
Measure 4: addressing continued supply from the lake bed
Dredging has reduced continued supply of phosphate[3,6] by 60 per cent, from an average of 3.5 to 1.5 milligrams P/m2/d. To ensure that phosphate is no longer released from the solid lake bed, Phoslock was used. Phoslock is lanthanum-modified clay, which binds and sequesters residual phosphate in the water column and the residually released phosphate.
Measure 5: management and maintenance
The load on the water system has been reduced by removing overhanging branches and some of the many leafy trees directly along the water. Run-off of seepage and other water from the dike is limited by a raised footpath along the pond.
Reduced intake of water naturally leads to a decrease in the external phosphate load the lake has to deal with (a decrease of almost 2 mg P/m2/d). On the other hand, the water spends considerably more time in the lake, lowering the critical phosphate load. In addition, dredging led to a slightly increased water depth, also resulting in a reduction of the critical phosphate load. Thus, the upper critical limit ('from clear to turbid') dropped from 5.0 to 2.9 milligrams P/m2/d. A recalculation taking into account the effect of all measures shows that the external phosphate load is now around this upper critical limit[8].
Before the measures, there were seasonal fluctuations in phosphate concentrations. Concentrations were higher in summer than in winter due to continued supply from lake bed sediment. After the measures, these fluctuations disappeared (Figure 1). Total nitrogen concentrations have also fallen, and large fluctuations have failed to materialise. This has greatly reduced the amount of algae (Figure 2).
The measures have greatly improved conditions for submerged aquatic plants, resulting in a spectacular increase in the number of plants covering the lake bed. Annual monitoring shows that this submerged vegetation has increased from an average of 30 per cent in 2018 to an average of 85 per cent in 2022. In 2022, Elodea nutallii (western waterweed) and several Chara species (stoneworts) were the dominant species.
It will take time for the measures to fully impact the ecosystem. We are seeing positive developments in monitoring of bathing water. Cyanobacteria blooms can never be completely prevented, but the chances of them happening are now low. No negative bathing advice was issued in 2020–2022 for cyanobacteria or bacteriological contamination.
WFD monitoring shows that the ecology is responding positively to the new conditions. Algae are decreasing, aquatic plants are increasing and fish stocks are slowly adapting.
The intervention in Bleiswijkse Zoom shows that it is possible to restore an ecosystem from a turbid, algae-rich state to a clear state with abundant aquatic plants. The systems analysis provided the rationale for measures aimed at reducing external and internal nutrient loads and increasing the ecosystem’s ability to support life. Bleiswijkse Zoom is a watery area and greenbelt with an officially designated bathing site. For years, the bathing site struggled with cyanobacteria blooms during the bathing season and the associated negative bathing advice. To improve bathing water quality and meet WFD targets, an integrated plan has been drawn up based on a systems analysis using the ecological key factors. The measures were aimed at reducing nutrient loads and increasing the ability to support life. This approach appears to be working: nutrient concentrations have dropped considerably and aquatic plants have increased significantly. In the new situation, Bleiswijkse Zoom already almost meets the WFD targets. With further natural development, water quality is expected to meet all WFD targets in the foreseeable future.
Nutrient concentrations decreased significantly, plant growth increased, the water became clearer and bathing water quality improved. The Schieland en de Krimpenerwaard Water Authority continues to monitor ecological development and may apply conservation management in the future if necessary. Bleiswijkse Zoom now almost meets the WFD targets, and our expectation is that, with further natural development, all WFD targets will be met.
Marit Meier
(Schieland en de Krimpenerwaard Water Authority)
Anne Rutgrink
(Schieland en de Krimpenerwaard Water Authority)
Vincent Blom
(Schieland en de Krimpenerwaard Water Authority)
Jeroen Mandemakers
(Witteveen+Bos)
Miquel Lurling
(Wageningen University & Research)
Said Yasseri
(Limnological Solutions International)
Application of Phoslock in Bleiswijkse Zoom. Photo JP Schilder bv
Summary
1. Witteveen+Bos (2013). Systeemanalyse recreatiewateren (Systems analysis of recreational waters). Report no. RT722-1
2. Stowa (s.a.). Ecologische Sleutelfactoren voor stilstaande wateren (Ecological Key Factors for stagnant waters). Retrieved from www.stowa.nl, consulted 6 May 2024
3. Smolders, F. & van Mullekom, M. (2015). Bodem- en Hydrochemisch onderzoek Bleiswijkse Zoom (Soil and Hydrochemical Survey at Bleiswijkse Zoom). B-WARE
4. Niemeijer, B. & Mies, J. (2018). KRW visstandonderzoek HHSK (Schieland en de Krimpenerwaard Water Authority WFD fish stock survey). ATKB
5. Kampen, J. & Kleppe, R. (2021). Eindrapportage afvissing Bleiswijkse Zoom (Final report on removing fish from Bleiswijkse Zoom). ATKB Waardenburg Report Number 20180840/rap02
6. Yasseri, S. & Mucci, M. (2019 and 2020). Bleiswijkse Zoom, Releasable Phosphorus from Bleiswijkse Zoom Sediments Assessment for a Phoslock application. LSI-Limnological Solutions International, report for HHSK
7. Janse, J.H. et al. (2010). Estimating the critical phosphorus loading of shallow lakes with the ecosystem model PCLake: sensitivity, calibrations and uncertainty. Ecological Modelling 221(4):654-665.
8. Witteveen+Bos (2021). Update water- en stoffenbalans Bleiswijkse Zoom (Bleiswijkse Zoom water and substance balance update).
9. HHSK (2023). Waterkwaliteitsrapportage 2022 (Water Quality Report 2022.).
10. HHSK (2023). Rapportage Bleiswijkse Zoom (Bleiswijkse Zoom Report)
^ Back to start
Ecosystem restored
Many nature-friendly banks (NFB) are being constructed in the Netherlands to improve the ecological water quality of ditches and canals. At some of these sites floating pennywort is present. This is a non-native, invasive aquatic plant species. It grows from the bank onto the water. Under favourable growing conditions, floating pennywort has a very high growth rate and can overgrow native aquatic plants and even the entire watercourse. Does the construction of a NFB further facilitate floating pennywort?
Floating pennywort can negatively affect an ecosystem[1], but it remains unclear what would happen to the ecological quality of a watercourse and its banks when a nature-friendly bank (NFB) is constructed at sites with floating pennywort.
We selected watercourses where floating pennywort was already present and where the NFB had been constructed several years earlier. This ensured that the vegetation has been able to develop for several years. The project did not run long enough to monitor the development of new NFBs.
Four types of NFB were studied: very gently sloped NFBs, moderately gently sloped NFBs, steep NFBs and shallow marsh NFBs (a type of NFB that creates a zone of shallow water parallel to the watercourse; ‘plasberm’ in Dutch). Each surveyed NFB-transect was compared to a nearby reference transect of a watercourse in the same area of which the bank has not been restored (both transects about 100 m long; both riparian and aquatic zones studied). As the largest difference between the pairs (NFB vs reference) was expected in very gently sloped NFBs, these bank types were sampled the most.
To test the difference between NFB and reference, paired t-tests were performed on the monitoring data of the abundance of floating pennywort and of ecological quality. To test whether the abundance of floating pennywort and ecological quality differed among types of NFBs, repeated measures ANOVA was performed. Only a few transects were available for each bank type individually (Table 1), which makes the results of the ANOVA test more uncertain.
Ecological quality was determined using the following parameters: the Ecological Quality Ratio of the aquatic plants (aquatic plant EQR of the EU Water Framework Directive (WFD), based on plant species composition of the water and riparian zone, and cover by growth form), the QuickScanScore for macrofauna (QS score, based on a number of indicative macrofauna groups), the number of plant species in the water and riparian zone, the number of macrofauna families and the amount of floating pennywort. Since vegetation can vary in species composition, cover and height over time, Aquon surveyed the vegetation at three separate times over two years: in August 2022 and in June and September 2023. In the first year, ten NFBs with corresponding references were studied, to which three very gently sloped NFBs with references were added in the second year, see Table 2[2].
Vegetation
The total number of plant species was significantly higher only at very gently sloped NFBs compared to their references (paired t-test, Table 1). This is largely due to the number of plant species found in the riparian zone; on average, the number of aquatic plant species in the aquatic zone didn’t differ between NFB and reference transects. For all other NFB types, there was no significant difference in the quality variables between NFB and reference transects. The average amount of floating pennywort on the NFB transects also did not significantly differ from the amount on the reference transects (paired t-test; Table 1).
In addition, the average amount of floating pennywort was not statistically significantly different among the NFB types (ANOVA analyses; p>0.05). Aquatic plant EQR, plant diversity and macrofauna QS-score also did not differ significantly among NFB types. Note that the number of sampled sites per NFB type was limited for these ANOVA analyses.
We found that floating pennyworth cover was limited in most of the studied transects. One area stands out though, because of the relatively large amount of floating pennywort (transects numbers 6.1–6.4 in Table 2). In this area, all NFB types except a very gently sloped NFB were present. The amount of floating pennywort was higher at the moderately gently sloped bank and at the marsh NFB, than at their reference transects. However, the number of plant species in the aquatic and riparian zones was still more or less similar (quite low) among all NFB and reference transects.
A positive value means an average increase of the variable at the NFB compared to its reference. A negative value (orange) means an average decrease. If a cell is coloured in green, this indicates a significant difference between NFBs and their reference (p < 0.05). No statistical testing was possible for the marsh NFB, as there was only one of this type sampled in the study.
Most of the transects surveyed did not score well on ecological quality (Table 2), which can not entirely be contributed to the presence of floating pennywort. Transects with a low aquatic plant EQR often harboured a dominant, disturbance-tolerant plant species such as reed that can also reduce aquatic plant EQR when very abundant.
Macrofauna
For the macrofauna Quick Scan score and the number of macrofauna families sampled in this Quick Scan, no statistically significant difference was found between the NFB and their reference transects (paired t-test p>0.05). The macrofauna QS score was higher for steep than for very gently sloped NFBs (ANOVA, p=0.00095). The same is true for the number of families (ANOVA, p=0.00069). This does not seem to be directly attributable to the presence of floating pennywort. The positive correlation between the macrofauna QS score and the presence of floating pennywort explains only a minimal fraction of the variation of the QS score (R2 = 0.0405).
In general, all studied sites score 'moderate' to 'poor' in the macrofauna Quick Scan (Table 2). The reason for this has not been investigated within this project.
It is striking that the aquatic plant EQRs of the same transects can differ by an entire EQR class among the different sampling moments (Table 2). The QS score differs less over time than the aquatic plant EQR, but even the QS-score of some transects fall into different QS classes at different sampling moments. One explanation could be that weather was more favourable for the positive indicator species in 2022 than in 2023, another is the variation within transects was the result of factors such as succession, management or the seasonal timing of the sampling. Currently (spring 2024), Witteveen & Bos is conducting research on WFD methods on which our EQR and QS-score are based.
The first digit of the transect codes indicates the area code, the second the transect code. NA = not available. Areas 7 and 8 were not added to the project until 2023.
In this study, the amount of floating pennywort found on the NFBs was not greater than the amount found on their nearby reference transects (parts of the watercourse had not been restored). There was also no statistically significant difference in total number of plant species (except at very shallow NFBs), the aquatic plant EQR or the macrofauna Quick Scan score between the NFBs and their references. Almost all the sites investigated in this study (NFBs as well as references) did not score well on the aquatic plant EQR and the macrofauna Quick Scan score.
This study therefore does not demonstrate that floating pennywort benefits greatly from the construction of a NFB or from a specific bank profile, although a marsh NFB (‘plasberm’ in Dutch) is potentially risky (see transects 6.1–6.4). A very gently sloped NFB does create a large gradient from water to land, which is an advantage for riparian plant diversity (this study and national research[3]). The aquatic zone does not seem to benefit directly from an NFB in terms of ecological value.
Floating pennywort has always been managed at the locations of this study. It is not known how dominant floating pennywort would become without this regular management. Management with attention to removing floating pennywort, plant development in the aquatic zone and avoiding bare spots in the bank around the waterline is especially important and recommended. Floating pennywort fragments easily and is therefore good at colonising or recolonising open areas left, f.i. by intense management or bank restoration projects (such as the construction of NFBs)[4].
Based on our research, it is expected that construction of an NFB in these areas with floating pennywort will, on average, not lead to a negative effect on aquatic plant EQR, but will in itself not be sufficient to meet ecological targets. Floating pennywort thrives in nutrient-rich water[4]. Conditions such as good water and sediment quality and sound ecological management are therefore required as well.
Bank restoration, such as the construction of NFBs, could provide an opportunity to remove the invasive plant species and afterwards permanently suppress or even eliminate it using a sound management plan continuing for years after construction is completed (aftercare). However, more research is needed on this topic. At all times, ensure that management or construction of NFBs do not cause further spread of the species (via fragments).
Thanks to the De Dommel Water Board and the Aa en Maas Water Board for funding this study. We would also like to thank the Aquon and water board staff involved for their input. FLORON, together with water boards Aa en Maas and De Dommel, investigated whether the presence of floating pennywort detracts from the value of a nature-friendly bank (NFB). To this end, they looked at the effects on vegetation and macrofauna of NFBs with different degrees of steepness in watercourses with presence of floating pennywort. Over the course of 2 years, 13 pairs of NFBs and nearby reference transects of bank that had not been restored were examined. On average, there was no significant difference between the plant EQR and macrofauna QS scores of the NFBs compared to their references. More plant species were found on the NFBs with very gently sloped banks than on the banks of their reference. The study provides no clear evidence that construction of NFBs along a watercourse infested with floating pennywort would create additional risks in the surveyed areas, which generally had limited ecological quality. However, active management targeting floating pennywort is recommended.
Elske Koppenaal
(FLORON)
Michiel Verhofstad
(FLORON)
Max van de Ven
(De Dommel Water Board)
Bart Brugmans
(Aa en Maas Water Board)
Carlo Rutjes
(Aa en Maas Water Board)
Bram Spierings
(Aa en Maas Water Board)
De Dommel Water Board, nature-friendly bank Vossenbergweg
Summary
1. Hussner, A. E. & Lösch, R. (2007). Growth and photosyntheses of Hydrocotyle ranunculoides L. fil. in Central Europe. Flora 202: 653-660. https:// DOI:10.1016/j.flora.2007.05.006
2. Koppenaal, E.C. & Verhofstad, M. (2024). Grote waternavel: onderzoek naar kansen en risico’s in natuurvriendelijke oevers (Floating pennywort: investigating opportunities and risks in nature-friendly banks). FLORON report no. 2021.183.e1.
3. Verhofstad, M.J.J.M. et al. (2022). Kunstmatig natuurlijk. Een evaluatie van de meerwaarde van natuurvriendelijke oever (Artificially natural: An evaluation of the added value of nature-friendly banks). Floron report no. FL.2017.034.e3 – data: 2017 to 2021.
4. Walsh, G.C. et al. (2013). Persistence of floating pennywort patches (Hydrocotyle ranunculoides, Araliaceae) in a canal in its native temperate range: Effect of its natural enemies. Aquatic Botany 110: 78-83. https://doi.org/10.1016/j.aquabot.2013.05.011
^ Back to start
Impact nature-friendly banks
Previous editions
The knowledge section Water Matters of H2O is an initiative of
Royal Dutch Waternetwerk
Independent knowledge networking organisation for and by Dutch water professionals.
Water Matters is supported by
Alterra Wageningen University
Research institute that contributes by qualified and independent research to the realisation of a high quality and sustainable green living environment.
ARCADIS
Global natural and built asset design and consultancy firm working to deliver sustainable outcomes through the application of design, consultancy, engineering, project and management services.
Deltares
Independent institute for applied research in the field of water, subsurface and infrastructure. Throughout the world, we work on smart solutions, innovations and applications for people, environment and society.
KWR Watercycle Research Institute
Institute that assists society in optimally organising and using the water cycle by creating knowledge through research; building bridges between science, business and society; promoting societal innovation by applying knowledge.
Royal HaskoningDHV
Independent international engineering and project management consultancy that contributes to a sustainable environment in cooperation with clients and partners.
Foundation for Applied Water Research (STOWA)
Knowledge centre of the regional water managers (mostly the Water Boards) in the Netherlands. Its mission is to develop, collect, distribute and implement applied knowledge, which the water managers need in order to adequately carry out the tasks that their work supports.
Netherlands Water Partnership
United Dutch Water Expertise. A network of 200 Dutch Water Organisations (public and private). One stop shop for water solutions, from watertechnology to coastal engineering, from sensor technology to integrated water solutions for urban deltas.